16 December 2021
A Cold Pair of Mirrors Made Stable
A team of scientists from the Ludwig Maximilian University of Munich and attocube systems AG have engineered a Fabry-Pérot cavity at cryogenic temperatures under closed-cycle cooling conditions. The results have been published in PRX Quantum.
Studying the interactions between light and matter is fundamental for new developments in science and technology. Related experiments in solid state physics use devices called optical resonators to confine light to a small volume in space for a prolonged period of time. One method of creating an optical resonator is to position two mirrors facing each other at a close distance, calculated based on the optical wavelength, confining the light between the parallel surfaces. This kind of resonator is called a Fabry-Pérot cavity. Whereas certain kinds of cavities are fixed in position, an open Fabry-Pérot cavity allows the relative position of the mirrors to be adjusted in all spatial dimensions. Setups of this nature are, however, extremely sensitive. A team of scientists at the Ludwig Maximilian University of Munich and attocube systems AG, led by Prof. Alexander Högele and Prof. Khaled Karrai, now been able to engineer a Fabry-Pérot cavity at cryogenic temperatures within a closed-cycle cryostat. The results have been published in PRX Quantum.
Many experiments in solid state physics are performed at extremely low temperatures, which is achieved with machines called cryostats, which often use liquid helium to achieve those temperatures. However, there is a finite and dwindling supply of helium in the world, which will make these experiments more difficult and expensive over the next few decades. It is therefore expected that emerging quantum technologies will have to use helium more efficiently, which can be achieved using a “closed-cycle” cryostat. These machines use far less helium overall, since they use helium gas instead of liquid helium, and it is efficiently recycled within the closed-loop system.
However, using closed-cycle cryostats comes at the cost of increased mechanical vibrations, which can make certain delicate experiments much more difficult. In particular, this brings a major challenge for light-matter interaction experiments: the mirrors of an open Fabry-Pérot cavity must be positioned with high accuracy and stability in order for the efficient confinement of photons. The quality factor of an optical resonator is a parameter that indicates how long the photons are confined inside the cavity; a quality factor of one hundred, for example, means a photon will stay in the cavity a hundred times longer than it would when traveling the same distance outside of the cavity.
Despite these significant challenges, Prof. Högele and Prof. Karrai’s team were to engineer an open Fabry-Pérot cavity inside a closed-cycle cryostat, which still provides significant tunability and high mechanical stability. Their system consists of a planar (flat) mirror, which can serve as a host for solid-state emitters – in this case the transition-metal dichalcogenide semiconductor WSe2 – and an optical fiber with a concave tip. (This fiber, seen at the bottom of the figure, was developed in the research group of Prof. David Hunger at the Karlsruhe Institute of Technology.) With a combination of passive and active vibration reduction techniques, the fluctuations in the cavity length were reduced down to root mean square value of less than 100 picometers. This represents an improvement by more than five orders of magnitude compared to the typical mechanical vibrations induced by a closed-cycle cryostat. This stability enables experiments with cavity quality factors of up to 1 million.
Using this novel platform, the researchers demonstrated efficient light-matter interaction with a two-dimensional monolayer of transition metal dichalcogenide semiconductor WSe2. The material is representative of the class of atomically thin semiconductors with a unique interface between photons and spin-valley polarized excitons, which have a wide range of applications in the emerging field of opto-valleytronics. In this study, excitons were made to interact with photons inside the cavity in the regime of strong (very efficient) light-matter interaction, enabled by the high quality factor of the open cavity and the reduced temperatures in the cryostat, leading to the emergence of hybrid light-matter quasiparticles called exciton-polaritons.
Next, the research team plans to use the cavity setup to explore light-matter interaction in newly emerging semiconductor van der Waals heterostructures – composites made from multiple two-dimensional layers – hosting hybrid moiré excitons. Scientists can spatially pin and manipulate excitons leading to novel optical and electronic properties. In the broad scope of light-matter interaction in solid-state systems, the high cavity stability achieved by the team will facilitate a wide range of cavity quantum electrodynamics experiments with other solid-state materials, like quantum dots and quantum wells in conventional semiconductors, color centers, or rare-earth ions.
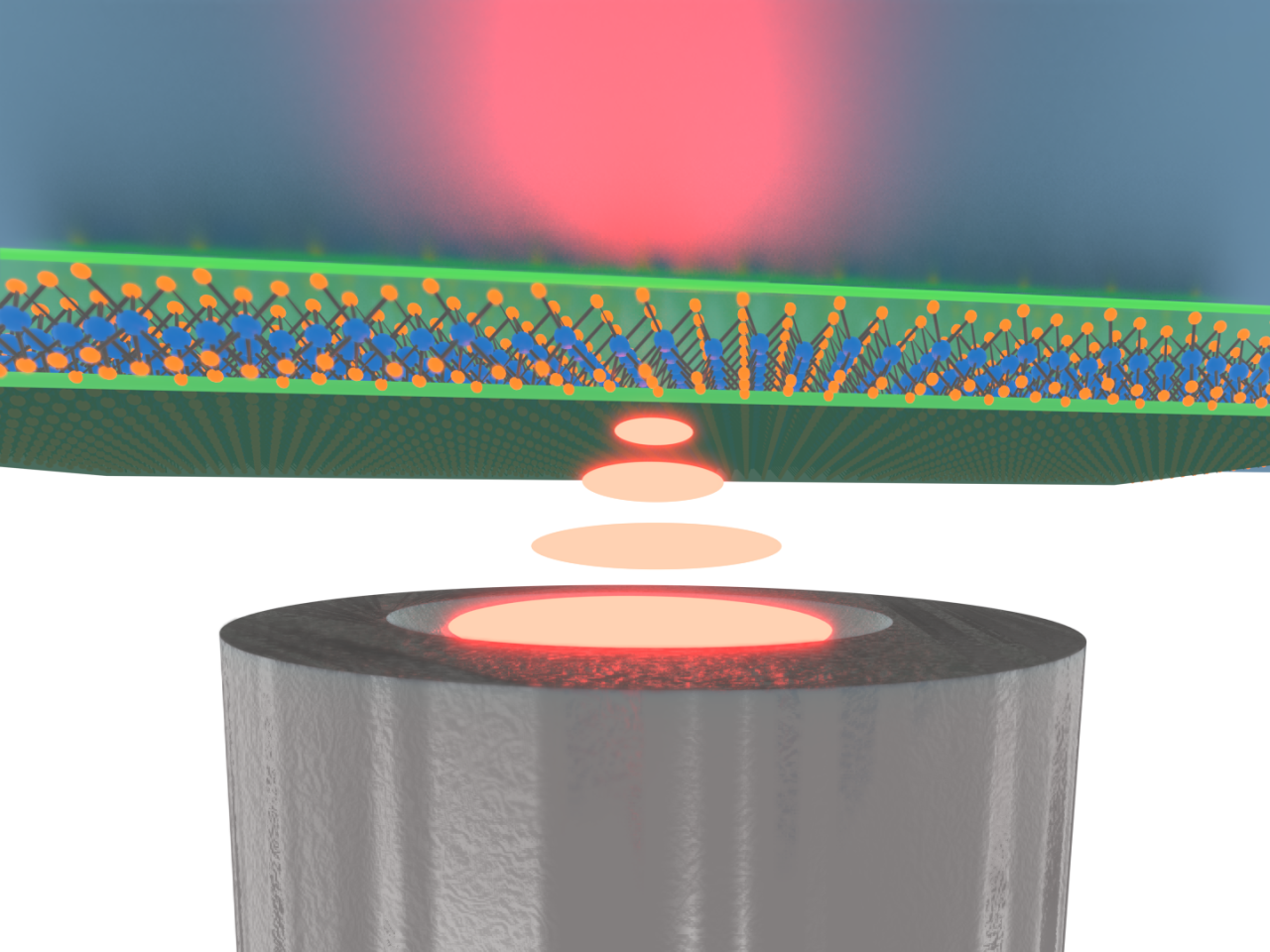
Figure: At the bottom is a tip of an optical fiber with concave depression profile, about 125 micrometers across. This concave profile helps guide the light, making confinement volume as small as possible when it interacts with the material. The gray surface at the top is the mirror. The material attached to the mirror is transition-metal dichalcogenide semiconductor WSe2, composed of tungsten (blue) and selenium (orange) atoms.
Publication
Open-Cavity in Closed-Cycle Cryostat as a Quantum Optics Platform
Samarth Vadia, Johannes Scherzer, Holger Thierschmann, Clemens Schäfermeier, Claudio Dal Savio, Takashi Taniguchi, Kenji Watanabe, David Hunger, Khaled Karraï, and Alexander Högele
PRX Quantum, 27 Oct 2021.
DOI: 10.1103/PRXQuantum.2.040318
Contact
Prof. Dr. Alexander Högele
Ludwig-Maximilians-Universität München
alexander.hoegele[at]lmu.de
Dr. Samarth Vadia
Ludwig-Maximilians-Universität München
samarth.vadia[at]physik.lmu.de