15 February 2023
Matchmaking atoms into a new molecular Fermi gas
Researchers at MPQ and MCQST have created a first-ever degenerate Fermi gas of sodium-potassium molecules. To this end, they drove a Bose-Fermi atomic mixture through a previously hypothetical quantum phase transition. The team's trick is to match the densities of a Bose-Einstein condensate (BEC) of sodium atoms and a Fermi gas of potassium atoms. This allows to reduce the losses from collisions of the atoms during the reaction process, which so far had been a major bottleneck for creating degenerate Fermi gas of molecules. The work is an important step towards the generation of large and stable quantum gases of polar molecules and paves the way for a better understanding of strongly interacting Bose-Fermi atom mixtures.
About three decades ago, physicists succeeded for the first time in producing a quantum-degenerate gas of atoms: a so-called Bose-Einstein condensate. Since then, researchers have been struggling to cool down ground-state molecules to such an extent that they exhibit quantum-degenerate properties. For example, these ground-state molecules have a large dipole moment and many internal degrees of freedom, which makes them well suited for use in quantum simulations, quantum computers or for chemical processes at ultra-low temperatures. In addition, fundamental physical symmetries could be tested with them. However, due to the complex structure of molecules, it is difficult to cool them down in a direct way. Therefore, a team of theoretical and experimental researchers at the Max Planck Institute of Quantum Optics (MPQ) in Garching relied on a different approach to obtain quantum-degenerate ground state molecules.
The bottleneck: dense bosons and dilute fermions
The scientists led by Dr. Xin-Yu Luo, head of the Laboratory for Ultracold Polar Molecules in the Department of Quantum Many-Body Systems, reversed the order of the procedure and assembled the molecules from atoms that they had previously cooled into the quantum degenerate range. However, this was not enough. "Although we took advantage of powerful cooling techniques for atoms, the remaining challenges in assembling atoms were greater than we expected," Luo reports: "both in experiment and in terms of theoretical understanding."
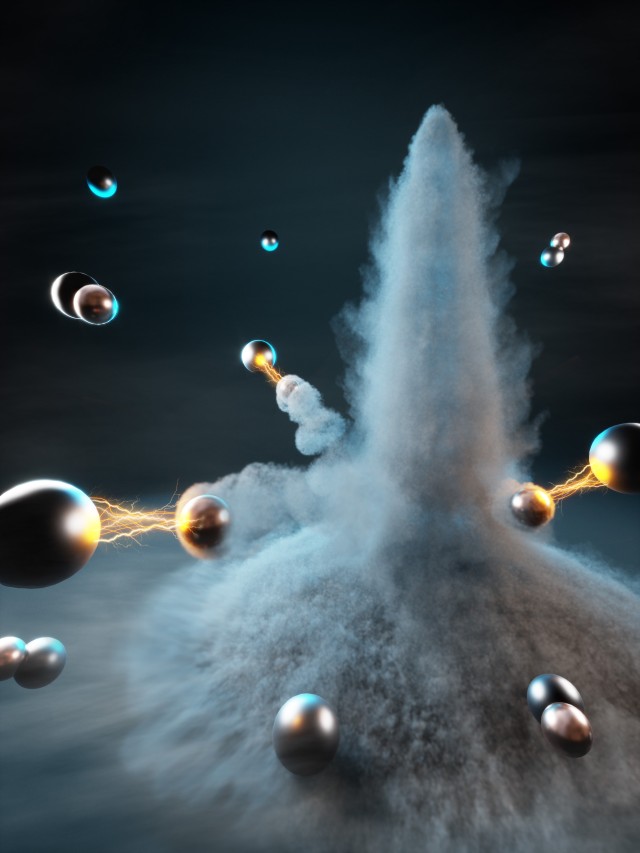
On the theory side, it has been discussed for nearly two decades how a mixture of a Bose-Einstein condensate and a Fermi gas can nevertheless be efficiently associated to form molecules. Simplified models that only took into account the interaction of two particles predicted a vanishing efficiency at a temperature of zero degrees, which spoken in terms of theoretical physics is due to a small overlap of bosons and fermions in phase space. "Therefore, the joint action of many atoms is necessary to allow efficient formation of molecules in a Bose-Fermi mixture near absolute zero," Luo concludes.
Large molecular fermi gas from density-matched atoms
The team at MPQ has been working for more than a decade to produce a degenerate gas of sodium-potassium molecules. "For a long time, we failed to do so because of the strong collision losses in doubly degenerate mixtures," reports Dr Marcel Duda, a former PhD student in Xin-Yu Luo's team. Therefore, we systematically investigated different collisions between atoms and molecules to find out where the bottleneck lies. Based on these findings, the researchers were able to develop a simple technique to reduce the density of the BEC and match it to the density of the Fermi gas using a dipole trap that confines sodium atoms much more weakly than potassium atoms. "This allows us to eliminate the losses and thus associate 80 percent of the Bose-Einstein condensate into molecules," says Duda. The temperature of these so-called Feshbach molecules is well below the Fermi temperature, where quantum effects begin to dominate. "So, while we struggled to get into the quantum-degenerate regime, as did many other labs, the density matching solution is quite simple," Duda enthuses. "Now we can routinely make 50,000 to 70,000 sodium-potassium Feshbach molecules." In a final step, using the so-called stimulated Raman adiabatic passage technique these Feshbach molecules are then converted to their ground state, in which they exhibit a large permanent dipole moment.
A good benchmark for further modelling
"The near 100 percent association efficiency clearly shows us that the assembly of a doubly degenerate Bose-Fermi mixture cannot be a two-body process," Xin-Yu Luo sums up. Instead, our measurements agreed well with a model that predicts a quantum phase transition from a polaronic to a molecular phase. "If the attraction between bosons and fermions is weak, the mixture can be understood qualitatively as a condensate of Fermi polarons – quasiparticles in which the interaction between the bosons and the fermions surrounding them leads to a kind of cloud around the bosons," explains Jonas von Milczewski, a doctoral student in the theoretical research group at MPQ led by Prof. Dr. Richard Schmidt. The condensate thus disappears near the predicted critical strength of the interaction, at which all bosons combine with the fermions to form molecules. As a result, the system turns into a Fermi gas of Feshbach molecules. "The agreement between our measurements and the model provides an important benchmark for modelling strongly interacting, doubly degenerate Bose-Fermi mixtures," Luo notes.
This could bring physicists closer to answers to many unanswered questions about the underlying quantum phase transition. These include the question of the order of the phase transition as well as hysteresis effects and the dynamics during the transition. Since its critical point depends on density, the signal near the critical point is blurred by the different densities of the mixture used by Xin-Yu Luo and his team, which is trapped in a harmonic trap. The researchers now want to overcome this obstacle by using a so-called box potential. "If you trap a mixture of millions of atoms in a box trap, you can also create a much larger Fermi gas consisting of about a million molecules," says Prof. Dr. Immanuel Bloch, Director of MPQ's Quantum Many-Particle Systems department. "This can be an excellent initial condition for evaporative cooling of molecules to a deeply degenerate regime which would facilitate the study of strongly interacting dipolar quantum gases."
Source: MPQ Website
Publication
Transition from a polaronic condensate to a degenerate Fermi gas of heteronuclear molecules
M. Duda, X.-Y. Chen, A. Schindewolf, R. Bause, J. von Milczewski, R. Schmidt, I. Bloch & X.-Y. Luo
Nature Physics (2023)
DOI:
10.1038/s41567-023-01948-1
Contact
Dr Xinyu Luo
xinyu.luo[at]mpq.mpg.de
Max Planck Institute of Quantum Optics, Garching
Prof. Dr Immanuel Bloch
Director
immanuel.bloch[at]mpq.mpg.de
Max Planck Institute of Quantum Optics
Katharina Jarrah
PR and Communications
katharina.jarrah[at]mpq.mpg.de
Max Planck Institute of Quantum Optics